植物天然农药除虫菊酯的生物合成和应用研究进展
1.
2.
3.
Biosynthesis and application of pyrethrins: a natural pesticide from plants
1.
2.
3.
收稿日期: 2021-06-21 修回日期: 2021-08-17 网络出版日期: 2021-11-19
基金资助: |
|
Received: 2021-06-21 Revised: 2021-08-17 Online: 2021-11-19
作者简介 About authors
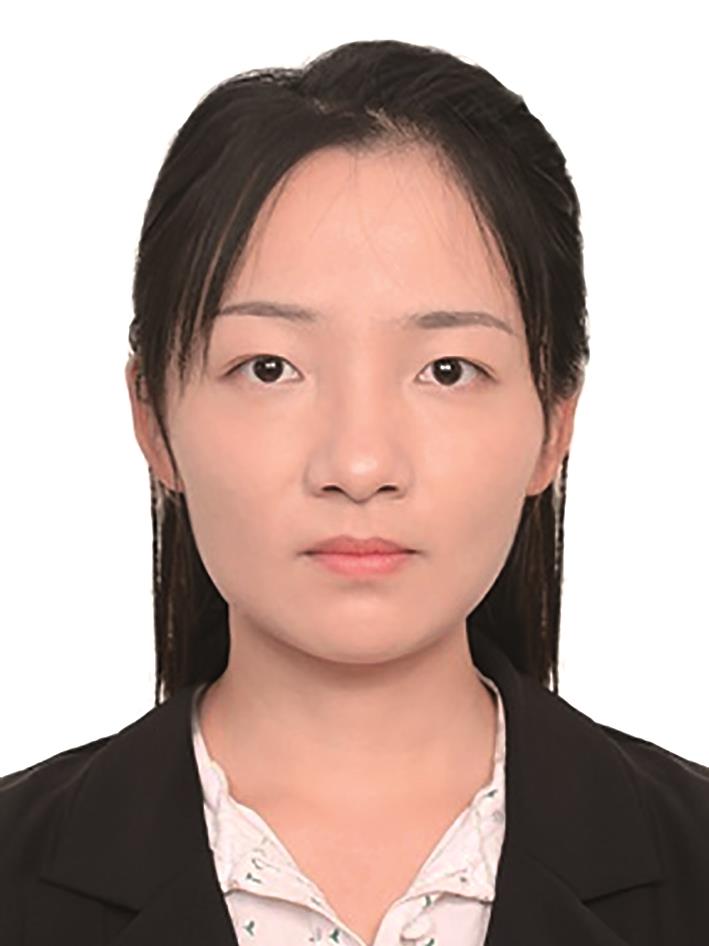
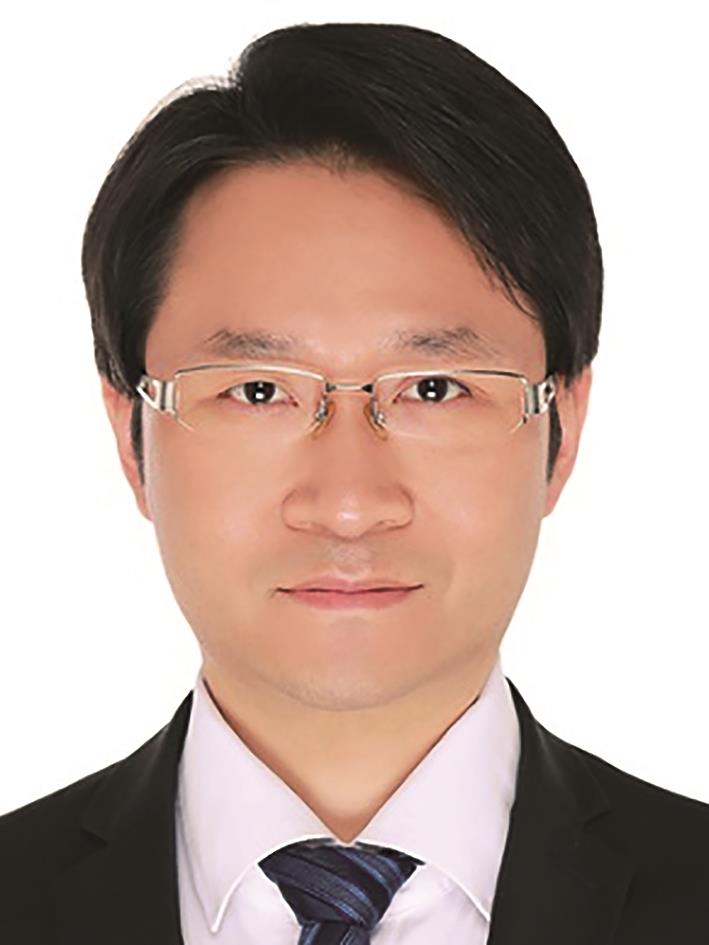
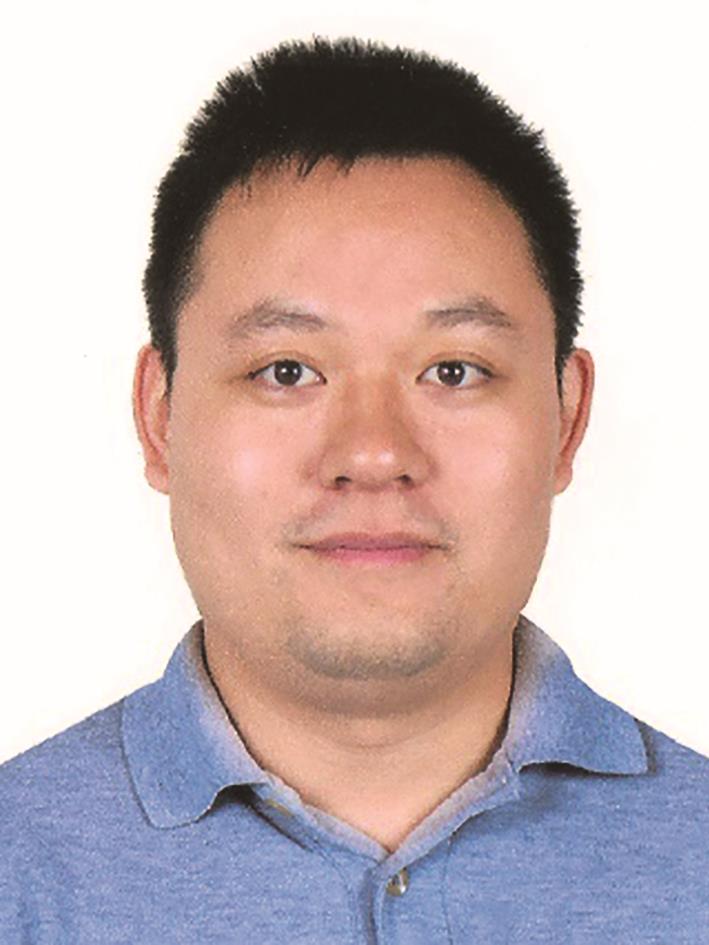
利用生物底盘进行生物农药的绿色低耗能生产是合成生物学未来发展的重要方向。除虫菊酯是一种源自菊科植物除虫菊的天然高效杀虫剂,具有广谱和强力的杀虫和驱虫作用,相较于化学合成的类似物(拟除虫菊酯),对哺乳动物毒性小,无环境危害,是生物农药的最优选择之一,具有广阔的应用前景。天然除虫菊酯含有六种主要成分,由两种异型萜类酸配体和茉莉酸合成途径来源的三种醇配体缩合而成。本文总结了除虫菊酯的研究历程,重点介绍了其生物合成途径解析与生物制造等方面的进展,综述了近期解析的细胞色素P450等相关生物合成酶,并讨论除虫菊酯生产中涉及的调控、转运和底盘适配等尚待解决的问题。随着合成生物学技术的发展,利用已解析的代谢合成途径在微生物等底盘表达体系规模化生产除虫菊酯,能够为合成生物学生产绿色生物农药的科学理论与应用实践提供重要范例。
关键词:
How to produce safe pesticides in chassis through energy-saving and environmentally friendly methods is one of challenges for synthetic biology. As a natural botanical insecticide, Pyrethrins derived from Pyrethrum (Tanacetum cinerariifolium) have good insecticidal and deworming activities with broad spectrum. Compared with chemical synthetic counterparts (pyrethroids), pyrethrins are less toxic and harmful to environment and human. These characteristics make pyrethrins not only one of the most ideal biological pesticides so far with broad applications and great potentials, but also candidates for their production via synthetic biology in the future. In this article, we review the discovery and chemical and biological characteristics of pyrethrins as well as the elucidation of their biosynthetic pathway by summarizing the catalytic enzymes identified recently including cytochrome P450s, dehydrogenases, methyltransferase and phosphatase etc. The synthesis process is mainly involved three parts: the synthesis of acid moieties, the synthesis of alcohol moieties and the condensation of acids and alcohols to esters. At present, the synthesis of acid moieties has been largely deciphered, and genes encoding key enzymes for acid-alcohol condensation have also been determined, but the synthesis of alcohol moieties needs to be explored further. With the identification of a large number of gene elements and the function of related genes in the plants, the rise of synthetic biology and the progress of synthetic technology, it is possible to express heterologous genes in chassis, integrate the pathway of heterologous synthesis to produce targeted products. This also makes it possible to express the metabolic pathway for the production of pyrethrins and their derivatives in suitable microbial chassis. Therefore, this review also focuses on challenges that need to be addressed in this regard, including regulation, transportation and chassis adaptation for more efficient production, and forecasts future development. We hope that more research progress could provide a solid scientific basis and application guidance for the biosynthesis of the green and effective pesticide, and finally realize the large-scale production of pyrethrins.
Keywords:
本文引用格式
王凤姣, 徐海洋, 闫建斌, 李伟.
Fengjiao WANG, Haiyang XU, Jianbin YAN, Wei LI.
农药对于农业生产至关重要,全球农药市场总体趋势呈现增长态势,自2018年开始全球杀虫剂市场以5.2%的年复合增长率持续增长,预计2025年全球杀虫剂市场销售额将达到276.1亿美元(
除虫菊酯是一种广泛使用的植物源杀虫剂,来自菊科植物除虫菊(Tanacetum cinerariifolium),是除虫菊花精油的主要成分。目前从除虫菊中天然提取仍然是除虫菊酯的主要生产方式,然而除虫菊的种植受海拔、土壤、气温的影响较为明显,全球每年的产量仅为0.8万吨,而对天然除虫菊酯的需求已超过2.1万吨,供给严重不足(
21世纪以来,合成生物学领域迅速发展,除虫菊酯作为一类重要的绿色杀虫剂,从简单的单酶催化到将合成元件流水线式组装入微生物细胞工厂或者是植物底盘,从头合成或从中间体通过一系列反应最终得到产物或者提高除虫菊酯在绿色农业上的应用,运用合成生物学理念,改变传统的除虫菊酯的应用方法,将有利于降低生产成本和环境污染。本文主要介绍了除虫菊酯的成分和结构、研究历史,阐明目前的生物合成机制,总结了目前天然除虫菊酯在合成生物学领域应用研究进展,期待在未来可以利用合成生物学手段提高绿色农药的利用效率。
1 除虫菊和除虫菊酯的生物合成
1.1 简介和研究历史
图1
图1
除虫菊酯发展与应用年鉴
Fig. 1
Chronicles for the development and application of pyrethrins and their derivatives
1.2 结构和分类
1923年,Yamamoto首次报道除虫菊中活性成分的化学结构含有一个环丙烷环[45]。1924年,诺贝尔奖获得者Staudinger和Ruzicka确定了酸配体部分的正确结构[46]。LaForge和Barthel于1944年最终确定了除虫菊酯Ⅰ和Ⅱ的正确结构,同时从除虫菊提取物中分离出瓜菊酯Ⅰ和Ⅱ[47]。除虫菊酯Ⅰ酸配体的绝对构型由Crombie和Harper于1954年确定[45],随后,除虫菊酯Ⅱ酸配体的绝对构型由Inouye和Ohno确定[45,48]。1958年,Katsuda和Inouye证实了醇配体的绝对构型,即除虫酮醇和瓜菊酮醇[49]。1966年,Godin和他的同事分离出两种更相关的次要成分,茉莉菊酯Ⅰ和Ⅱ(图2)[50]。这进一步促进了除虫菊酯作用方式和合成通路的研究。
图2
除虫菊可以合成六种不同的除虫菊酯,分为Ⅰ型和Ⅱ型的茉莉菊酯、除虫菊酯和瓜菊酯。从结构方面而言,除虫菊酯由一个醇配体和一个酸配体缩合而成[51]。依据侧链的不同,酸配体有两种:菊酸(chrysanthemic acid)和第二菊酸(pyrethric acid)。含有菊酸的为Ⅰ型,含有第二菊酸的为Ⅱ型。醇配体有三种,包括:瓜菊酮醇(cinerolone)、茉莉酮醇(jasmolone)和除虫菊酮醇(pyrethrolone),含有不同醇基的分别被命名为:瓜菊酯(cinerin)、茉莉菊酯(jasmolin)和除虫菊酯(pyrethrin),如图2所示[52]。不同除虫菊植株中6种除虫菊酯成分含量有所不同,除虫菊酯Ⅰ、Ⅱ占大部分(约70%),其含量直接决定了该混合物的杀虫活性[34]。
以天然除虫菊酯的结构为基础,对其酮醇部分或有机酸部分分别或同时进行修饰,合成相对更加稳定的拟除虫菊酯。根据其对不同配体的修饰不同,可以将其分为三类:对酸配体修饰所得的拟除虫菊酯(如四溴菊酯、氟胺氰菊酯等);对天然除虫菊酯醇配体修饰,如丙烯菊酯、炔呋菊酯等拟除虫菊酯;对醇、酸和酯键同时修饰,如醚菊酯、三氟醚菊酯等[53]。
1.3 合成途径
图3
图3
除虫菊酯生物合成途径
(除虫菊酯的配体合成起始于在除虫菊花器官的子房外壁腺体腺毛中,TcCDS催化2分子的DMAPP生成菊醇二磷酸,并在磷酸水解酶TcNudix1、脱氢酶TcADH2和TcALDH1的作用下生成菊酸,以及另外的氧化酶TcCHH和甲基转移酶TcCCMT的参与下形成第二菊酸;醇配体的前体茉莉酮通过茉莉酸的合成途径生成,而下游醇配体的合成由细胞色素P450酶TcJMH和TcPYS催化形成茉莉酮醇和除虫酮醇,瓜菊酮醇的合成途径尚不清楚;两种醇配体和三种酸配体在TcGLIP酶的催化下形成六种化合物。酸配体合成前期在质体中进行,后进入细胞质中进行进一步的氧化;茉莉酸的合成前体在质体中,后进入过氧化物酶体进行进一步反应,参与醇配体合成的细胞色素P450定位于内质网。分别定位于质体膜和过氧化物酶体膜的转运蛋白Jassy和CTS参与茉莉酸的合成,菊醇从质体中运出到细胞质和各配体从腺体腺毛运送到胞间是否需要转运蛋白还需要进一步验证)
Fig. 3
Pyrethrin biosynthesis pathway
(Synthesis of pyrethrin moieties are originated in the ovary trichome, TcCDS catalyzes 2 DMAPP to generate chrysanthemyl diphosphate, which is further catalyzed by phosphatase TcNudix1, dehydrogenases TcADH2 and TcALDH1 to generate chrysanthemic acid, and two additional enzymes oxidase TcCHH and methyltransferase TcCCMT participate the reaction to form pyrethric acid. Alcohol moieties are generated from the jasmonic acid biosynthesis pathway, and the downstream biosynthesis are catalyzed by cytochrome P450 TcJMH and TcPYS for the biosynthesis of jasmolone and pyrethrolone, but the reactions for cinerolone biosynthesis are still unknown. One of two acid moieties and one of three alcohol moieties are condensed by the catalysis of TcGLIP to produce six different pyrethrins. Upstream steps of the acid moiety pathway are located within plastid, and then the intermediates are transferred to cytosol for further oxidation. The biosynthesis of jasmonic acid is in the plastid and peroxisome under the catalysis of the cytochrome P450s localized at endoplasmic reticulum. Two transporter proteins Jassy and CTS involved in the biosynthesis of jasmonic acid are localized at the membrane of plastid and peroxisome, respectively, and more transporters may exist for transferring chrysanthemol from plastid to cytosol and also for transferring moieties from trichome to apoplast, which need further validation)
酸配体菊酸和第二菊酸来源于质体中1-脱氧-D-木酮糖-5-磷酸(DXP)萜类途径[1-deoxy-D-xylulose-5-phosphate(DXP)terpenoid pathway]。菊醇合成酶(TcCDS,chrysanthemyl diphosphate synthase)是除虫菊酯生物合成的第一个关键酶,定位于质体中,属于异型的萜类合成酶[15,22,55-56],能够催化两个二甲基烯丙基二磷酸(DMAPP,dimethylallyl pyrophosphate)分子生成菊醇二磷酸(CPP,chrysanthemyl diphosphate)[56],CPP可以在同样定位于质体的磷酸水解酶Nudix1的作用下水解生产菊醇二磷酸[57],推测可能还有其他磷酸酶参与菊醇的合成[11,57]。菊醇是合成菊酸和第二菊酸的分支点。菊醇随后在两步脱氢酶(TcADH2,alcohol dehydrogenase 2和TcALDH1,aldehyde dehydrogenase 1)的作用下,依次氧化为菊酮和菊酸[11];菊醇在羟化酶(TcCHH,chrysanthemol 10-hydroxylase)、脱氢酶TcADH2和TcALDH2的作用下生成10-羧基菊酸,然后进一步在甲基转移酶(TcCCMT,10-carboxychrysanthemic acid methyltransferase)的作用下将C10位氧化形成的羧基甲基化形成甲酯,即第二菊酸,这些步骤都发生在细胞质中[11]。
与除虫菊酯酸配体的生物合成相比,对醇配体的生物合成通路的解析尚不完整。用[1-13C]-D-葡萄糖饲喂产生除虫菊酯的除虫菊花器官,检测到与醇配体前体亚麻酸相一致的带有13C标记除虫菊酯[58-59]。两个细胞色素P450酶参与茉莉酮醇和除虫酮醇的合成,羟化酶(TcJMH,jasmone hydroxylase)催化茉莉酮(jasmone)生成茉莉酮醇开始,茉莉酮醇在除虫酮醇合成酶(TcPYS,pyrethrolone synthase)的作用下,将戊烯基的末尾碳碳键去饱和生成除虫酮醇[12-13,57]。上游前体茉莉酮,推测来自于茉莉酸合成途径,是以亚麻酸为前体的一系列氧化还原反应,合成最早发生在质体中,随后进入过氧化物酶体,分别定位于质体膜的JASSY和过氧化物体膜的CTS(COMATOSE)的两个转运蛋白参与中间体在不同细胞器间的运输。最近的标记研究表明,12-氧代植物二烯酸(OPDA)和顺式茉莉酮都是醇配体的前体,而反应绕过了茉莉酸[60],除虫菊中催化顺式茉莉酮生成的酶还需要进一步进行鉴定。
表1 参与除虫菊酯生物合成途径的基因
Tab. 1
基因 | 英文名称 | 中文名称 | 功能 | 文献 |
---|---|---|---|---|
TcAOS | allene oxide synthase | 丙二烯氧化物合酶 | 13-HPOT 12、13位C的氧化 | [62-63] |
TcAOC | allene oxide cyclase | 丙二烯氧化物环化酶 | 13-EOT生成OPDA(12-氧-植物二烯酸) | [62] |
TcOPR | 3-oxo-2-(2-pentenyl)-cyclopentane-1- octanoic acid reductase 3 | 12-氧-植物二烯酸还原酶 | OPDA 10、11位C还原 | [59, 64] |
TcJMH | jasmone hydroxylase | 茉莉酮羟化酶 | 茉莉酮4位C羟基化反应 | [12-13] |
TcPYS | pyrethrolone synthase | 除虫酮醇合成酶 | 茉莉酮醇戊烯基侧链去饱和 | [12] |
TcCDS | chrysanthemyl diphosphate synthase | 菊醇二磷酸合酶 | 酸配体骨架合成 | [22,56] |
TcNudix1 | nudix-family phosphatase | Nudix磷酸水解酶 | CPP去磷酸化 | [57] |
TcADH2 | alcohol dehydrogenase 2 | 醇脱氢酶 | 酸配体侧链氧化 | [11, 16] |
TcALDH1 | aldehyde dehydrogenase 1 | 醛脱氢酶 | 酸配体侧链氧化 | [11, 16] |
TcCHH | chrysanthemol 10-hydroxylase | 菊醇羟化酶 | 酸配体侧链羟化 | [15] |
TcCCMT | 10-carboxychrysanthemic acid 10- methyltransferase | 10-羧菊酸-10-甲基转移酶 | 10-羧基的甲基化 | [15] |
TcGLIP | GDSL lipase-like protein | GDSL脂肪酶 | 酸配体和醇配体缩合反应 | [51] |
TcLOX1 | lipoxygenase1 | 脂氧合酶 | 亚麻酸13位C的氧化 | [65] |
除虫菊酯生物合成在多个不同层面受到调控,包括组织和发育时期、细胞区隔和诱导积累,但是目前仅停留在基因表达和代谢物积累的测定上,尚未有分子生物学和生物化学的报道验证相关调控因子和转运蛋白,有待进一步研究。大部分生物合成发生在早期发育中的花蕾中转录分析表明,所有与除虫菊酯生物合成相关的基因主要在管状花中表达[11-13,15-16,57]。所有除虫菊酯生物合成基因的mRNA水平在花蕾发育早期都很高,但随着花的开放和成熟而减少[11,13,15-16,57]。除虫菊幼苗中可检测到低量的除虫菊酯,TcCDS等相关合成基因转录水平在幼苗中也相对较低[10,22]。除虫菊酯从花组织转移到发育中的瘦果中,并随后在胚中积累[10,22]。茉莉酸甲酯、挥发性有机化合物(VOCs,volatile organic compounds)和机械伤害都可以诱导菊酯在除虫菊花组织中的合成,且相关基因的表达和菊酯在营养组织中的积累均可以受到诱导[10,65-66]。除虫菊酯合成涉及多个细胞区室的转变,酸配体合成最早在质体,后转移至细胞质中,合成的酮醇配体也需要进入细胞间隙才会被定位其中的TcGLIP催化[22],尚不明确这些配体的转移是否需要转运蛋白的参与,如图3所示。
随着转录组共表达技术和各种体外验证手段,特别是烟草瞬时表达体系的成熟,菊酸和第二菊酸的完整合成途径,以及部分醇配体的合成途径得到解析。除虫菊的基因组草图近期也得到测定,大小为7.1 Gb,其中发现了大量的防御相关毒性蛋白、调控蛋白和代谢相关酶类的编码基因,有些为物种特异性基因[67]。以上条件为除虫菊酯合成途径的完全解析和调控研究奠定了基础,进而为利用合成生物学进行体外异源合成奠定了良好的基础。
2 除虫菊酯的合成应用
2.1 天然除虫菊酯传统生产方法的局限性
目前从除虫菊中提取仍然是天然除虫菊酯的主要生产方式,通常通过对磨碎的除虫菊花器官进行油基提取而获得,通过喷洒的方式使用。20世纪90年代之前,天然除虫菊酯的提取技术主要是溶剂萃取法,正己烷[68-69]、丙酮、甲醇、丙醇、二氯甲烷[69]、石油醚[70]和乙醇等有机溶剂进行提取[14, 71-72]。有机溶剂提取产能较快,但是涉及安全和污染的问题。随后超临界流体萃取工艺得到发展[73],其处理周期短,无溶剂回收问题,生产过程安全高效,加速了天然除虫菊酯市场的扩展。另外,微波萃取技术和超声波辅助提取以其工序短、节能低耗、溶剂少、安全稳定等优势,也得到了科研领域和技术市场的青睐[74-75]。天然提取的方法虽然可以大规模应用,但是受限于原料供应,生产量受到限制。
利用悬浮培养细胞进行代谢物的生产,能够确保在生产过程中更好地控制原材料的供应、质量和成本,而不受社会、政治、经济和气候波动的影响,所以高产愈伤组织细胞系产生除虫菊酯,可以作为一种替代品,成为除虫菊酯的原料库。Levy在1981年利用茎尖或者是叶芽作为外植体开发了一种成功的培养技术[76],但是除虫菊酯主要在花器官中合成,所以利用体外生产除虫菊酯存在一定的难度。1990年Zito和Tio分析了三年生温室植物的愈伤组织,结果表明,因为愈伤组织中亚麻酸的含量较低(在醇配体合成通路上),愈伤组织中产生的除虫菊酯含量较低[77]。Hitmi等培养的除虫菊愈伤组织产生的除虫菊酯的含量为1%左右,但是细胞悬浮培养不能积累除虫菊酯[78-79]。McLaughlin Gromley公司于1984年申请了一种用粗提的酶合成除虫菊酯专利。该生产方法制备含有除虫菊和万寿菊的无细胞匀浆并加入甲羟戊酸或焦磷酸异戊烯孵育产除虫菊酯[78]。目前悬浮细胞培养的方法在现实应用中并未得到推广。
2.2 除虫菊酯的异源表达合成进展
鉴于化学合成和离体培养的研究未能大规模应用,所以利用最新的合成生物学技术生产除虫菊酯成为一个新的研究方向。随着除虫菊酯合成途径的逐渐解析和底盘生物的构建成熟,利用合成生物学手段异源表达除虫菊酯合成酶,进行大规模生产除虫菊酯及其前体物质成为可能。由于途径的全面解析是最近几年才出现的进展,利用异源生物表达来进行合成生物学生产和改良作物抗性的相关研究尚处在起步阶段。
Hu等将除虫菊酯的酸配体第一个合成酶TcCDS(TcCHS)编码基因在杭菊(Chrysanthemum morifolium)中过表达,异源引入除虫菊酯的合成途径,结果检测到挥发性菊醇(volatile chrysanthemol)的释放和菊醇的糖基化衍生物菊醇苷(chrysanthemyl-6-O-malonyl-β-D-glucopyranoside)的积累,菊醇含量达到47 pmol/(h·g)(以鲜重计),菊醇苷的含量达到1.1 mmol/L,这两种成分对蚜虫具有独立的生物活性,且植株并没有导致有害表型的出现。TcCDS在植物中的表达显著减少了蚜虫的繁殖,诱导了一种双重防御系统,既有挥发性菊醇的气味驱避作用,又有其非挥发性糖苷对蚜虫产生的威慑作用[17]。
Xu等在番茄果实中重构了菊酸生物合成途径,该途径自然条件下产生高水平的四烯类色素番茄红素,这是一种与菊酸具有共同前体二甲基烯丙基二磷酸(DMAPP)的类异戊二烯类化合物,通过在番茄果实中表达来源于除虫菊的菊醇二磷酸合成酶(TcCDS)基因,和来源于野生番茄的醇脱氢酶(ADH)基因与醛脱氢酶(ALDH),实现了菊酸的异源生物合成。其中菊醇二磷酸合成酶基因来自于除虫菊,另外两个基因来自于野生番茄品种。表达这三种基因的番茄果实中的菊酸含量是非转基因植株中番茄红素的含量的1.7倍,达到67.1 μg/g FW,转移的DMAPP中有97%转化为菊酸[16]。
在随后的研究中,Xu等采用边解析边重构的策略,利用发掘的醇脱氢酶(TcADH2)、醛脱氢酶(TcALDH1)、细胞色素P450酶(TcCCH)和甲基转移酶(TcCCMT1)以及前期报道的TcCDS在烟草瞬时表达体系中完整重组了第二菊酸的合成通路,第二菊酸的总产量(包括游离和糖基化修饰)达到(24.0±2.7)μg/g[15],为异源合成除虫菊酯奠定了基础。
醇配体的异源重组合成尚未见报道,没有醇配体和酸配体的组装就无法形成完整的除虫菊配体。而且由于醇配体的前体茉莉酮在常见底盘物种(如烟草)中含量非常低,所以预测如果仅过表达参与合成的细胞色素P450,生成的产物含量也会过低没有应用价值。目前茉莉酮的合成途径也尚未完全解析,制约着代谢重组应用。茉莉酮的含量在很多植物(如茉莉花)的花器官中含量非常高,所以利用茉莉花等材料发掘茉莉酮的合成关键酶,是重组生产醇配体和完整除虫菊酯的必要条件。
目前除虫菊合成相关的调控因子和转运系统亦未有报道,关键催化酶及调控因子是否形成复合体亦未有报道,所以下游合成生物学应用受到限制,进一步深入研究除虫菊酯合成途径的调控和转运是除虫菊酯合成生物学应用的关键。未来更多的合成生物学技术和思路的引路将更进一步助力合成生物学的研究,包括但不限于:利用特殊的启动子优化组织特异性表达;通过密码子优化、增加基因的拷贝数和强启动子来增加节点基因的表达量;通过转运蛋白引入和亚细胞定位改造来聚合上下游代谢的空间区隔;催化酶的融合表达和蛋白质结构优化等手段增加催化活性;以及大片段组装多基因串联转化和表达来提高异源表达的效率等,均是合成生物学研究的重点。
3 展望
2016年美国农业部的报告预测,到2025年,生物基化学品的产值将超过5000亿美元,占全部化学品的25%左右[82]。设计和合成的工程细菌用于靶向治疗中的药物载体等。以青蒿酸异源合成为标志,合成生物学在天然产物、抗生素等的人工合成方面展现出巨大潜力。微生物发酵工程具有快速、便捷、易操控的优势,最新的发展通过多种技术将酶发掘、活性改造、途径和菌种优化、混菌发酵和发酵体系的工程优化等相结合,实现了酵母中阿片(opiate)类药物的全合成[83],丁醇生物的生产[84],青蒿酸[85]、紫杉烷类、硫醚抗生素等的合成[86]。植物源次级代谢产物在微生物中的人工生物合成,降低了对野生和珍稀植物资源的依赖,减少了对生态环境的破坏。目前还没有关于利用微生物工厂化生产除虫菊酯系列产物的研究和应用,随着其合成通路中关键酶得到进一步的挖掘,加强了利用微生物发酵工程进行工厂化生产的优势和潜力。
利用植物底盘进行除虫菊酯的生物技术生产具有重要意义,开发高效的植物底盘能够提高除虫菊酯的产量及其替代品的开发。与单细胞微生物相比,虽然植物体系更加复杂,周期更长、基因组更大、细胞器更多、代谢与调控机制更复杂,但是植物合成生物学研究也具有其独特的优势,植物底盘本身具有丰富的前体物质和完善的蛋白表达调控体系,植物来源的天然产物生物合成、调控及转运元件、线路和模块能更好适配植物底盘。在优化后植物底盘能够实现前体的足量供应和催化酶等的高表达,克服原产植物中特异性和含量低的缺点,实现在叶片、果实、毛状根、腺毛等组织中的规模化生产。同时作为自养生物,植物利用自然中的阳光、土壤、水等作为能量和营养的来源,是未来绿色合成生物学的一个重要研究方向。随着植物基因组、转录组、蛋白组、代谢组和表型组等组学大数据的快速发展,利用多种组学数据进行突变体库筛选、元件发掘以及模型预测加速了植物代谢网络和信号转导通路等数据发掘工作,已成为植物合成生物学研究的必备研究策略,为未来植物合成生物学研究提供了新的契机。
随着新型载体系统、工程菌、大片段组装和人工染色体以及高通量测序技术等的发展和完善,合成生物学的学科体系也日趋成熟。最近很多研究通过大片段组装,实现多个基因同时导入底盘细胞,实现目标产物的生产。例如Zhu等利用高效多基因转化叠加技术在胚乳中工程合成花青素培育出“紫胚乳水稻”[87];Fu等成功解析了紫锥菊中菊苣酸的生物合成途径,并在烟草中成功实现了异源构建[88]。随着除虫菊酯合成途径的解析,利用植物作为底盘进行除虫菊酯相关代谢产物的异源表达开始有了相关的报道[16],下一步通过大片段组装来进行除虫菊酯完整途径的构建和异源生产也具有更加广阔的前景,为未来新型绿色农药的开发和规模化生产提供保障,实现我国清洁、低能耗、无公害的农业产业发展。
参考文献
health
[M]//LARRAMENDY ML, SOLONESKI S.
Chemical pesticides and human health: the urgent need for a new concept in agriculture
[J].
Pesticides, environment, and food safety
[J].
Potential of microalgae as biopesticides to contribute to sustainable agriculture and environmental development
[J].
Glycyrrhiza glabra extract protects plants against important phytopathogenic fungi
[J].
Is the efficacy of biological control against plant diseases likely to be more durable than that of chemical pesticides
[J]
Methods to study the phytochemistry and bioactivity of essential oils
[J].
Botanical insecticides in the twenty-first century-fulfilling their promise
[J]
How plants synthesize pyrethrins: safe and biodegradable insecticides
[J].
Coexpression analysis identifies two oxidoreductases involved in the biosynthesis of the monoterpene acid moiety of natural pyrethrin insecticides in Tanacetum cinerariifolium
[J].
Pyrethrin biosynthesis: the cytochrome P450 oxidoreductase CYP82Q3 converts jasmolone to pyrethrolone
[J].
Jasmone hydroxylase, a key enzyme in the synthesis of the alcohol moiety of pyrethrin insecticides
[J].
Comparison of pyrethrins eextraction methods efficiencies
[J].
Pyrethric acid of natural pyrethrin insecticide: complete pathway elucidation and reconstitution in Nicotiana benthamiana
[J].
Production of trans-chrysanthemic acid, the monoterpene acid moiety of natural pyrethrin insecticides, in tomato fruit
[J].
Modification of chrysanthemum odour and taste with chrysanthemol synthase induces strong dual resistance against cotton aphids
[J].
Bugs in the system: insects, agricultural science, and professional aspirations in Britain, 1890-1920
[J].
Japan's pyrethrum position threatened
[J].
Pyrethrin from Dalmatian pyrethrum (Tanacetum cinerariifolium/Trevir./Sch. Bip.): Biosynthesis, biological activity, methods of extraction and determination
[J].
Pyrethrins protect pyrethrum leaves against attack by western flower thrips, Frankliniella occidentalis
[J].
Bidirectional secretions from glandular trichomes of pyrethrum enable immunization of seedlings
[J].
Chloride channels as tools for developing selective insecticides
[J].
DDT, pyrethrins, pyrethroids and insect sodium channels
[J].
Botanical insecticides: for richer, for poorer
[J].
Effectiveness of pure diatomaceous earth and different mixtures of diatomaceous earth with pyrethrins
[J].
Comparative bioactivity of selected extracts from Meliaceae and some commercial botanical insecticides against two noctuid caterpillars, Trichoplusia ni and Pseudaletia unipuncta
[J].
Investigating the potential of selected natural compounds to increase the potency of pyrethrum against houseflies Musca domestica (Diptera: Muscidae)
[J].
Biocompatible colloidal dispersions as potential formulations of natural pyrethrins: a structural and efficacy study
[J].
Use of pyrethrum in vector control
[J].
Impregnation of cotton fabric with pyrethrum extract in supercritical carbon dioxide
[J].
Nontarget effects of the mosquito adulticide pyrethrin applied aerially during a West Nile virus outbreak in an urban California environment
[J].
Pyrethrins and pyrethroid insecticides
[M]//LOPEZ O, FerNANDER-BOLANOS J.
Residue analysis and risk assessment of pyrethrins in open field and greenhouse turnips
[J].
Dissipation and residues of pyrethrins in leaf lettuce under greenhouse and open field conditions
[J].
Residue levels of pyrethrins and piperonyl butoxide in soil and runoff water
[J].
Residues and half-lives of pyrethrins on field-grown pepper and tomato
[J].
Residues and half-life times of pyrethrins on peaches after field treatments
[J].
Pyrethroid resistance in the pest mite, Halotydeus destructor: dominance patterns and a new method for resistance screening
[J].
Co‐occurrence of V1016I and F1534C mutations in the voltage‐gated sodium channel and resistance to pyrethroids in Aedes aegypti (L.) from the Colombian Caribbean region
[J].
Global occurrence of pyrethroid insecticides in sediment and the associated toxicological effects on benthic invertebrates: an overview
[J].
Agricultural insecticides threaten surface waters at the global scale
[J].
Distribution of Anopheles mosquito species, their vectorial role and profiling of knock-down resistance mutations in Botswana
[J].
Discovery and development of pyrethroid insecticides
[J].
Über Isolierung und Konstitution des wirksamen Teiles des dalmatinischen Insektenpulvers
[J].
Constituents of pyrethrum flowers; the partial synthesis of pyrethrins and cinerins and their relative toxicities
[J].
Studies on synthetic pyrethroids. Part V. synthesis of geometrical isomers of chrysanthemum dicarboxylic acid
[J].
The absolute configuration of naturally derived pyrethrolone and cinerolone
[J].
The jasmolins, new insecticidally active constituents of Chrysanthemum cinerariaefolium VIS
[J].
Identification and characterization of a GDSL lipase-like protein that catalyzes the ester-forming reaction for pyrethrin biosynthesis in Tanacetum cinerariifolium-a new target for plant protection
[J].
Total syntheses of all six chiral natural pyrethrins: Accurate determination of the physical properties, their insecticidal activities, and evaluation of synthetic methods
[J].
Progress and future of pyrethroids
[J].
Pyrethrum: a mixture of natural pyrethrins has potential for malaria vector control
[J].
Pyrethrin biosynthesis and its regulation in Chrysanthemum cinerariaefolium
[J].
Chrysanthemyl diphosphate synthase: isolation of the gene and characterization of the recombinant non-head-to-tail monoterpene synthase from Chrysanthemum cinerariaefolium
[J].
A trichome-specific, plastid-localized Tanacetum cinerariifolium nudix protein hydrolyzes the natural pyrethrin pesticide biosynthetic intermediate trans-chrysanthemyl diphosphate
[J].
Biosynthesis of pyrethrin I in seedlings of Chrysanthemum cinerariaefolium
[J].
Enzymes in jasmonate biosynthesis—structure, function, regulation
[J].
Elucidation of the biosynthetic pathway of cis-jasmone in Lasiodiplodia theobromae
[J].
Plant communication: Mediated by individual or blended VOCs
[J]
Allene oxide synthase, allene oxide cyclase and jasmonic acid levels in Lotus japonicus nodules
[J].
Functional characterization of an allene oxide synthase involved in biosynthesis of jasmonic acid and its influence on metabolite profiles and ethylene formation in tea (Camellia sinensis) flowers
[J].
An OPR3-independent pathway uses 4,5-didehydrojasmonate for jasmonate synthesis
[J].
Partial sequence isolation of DXS and AOS genes and gene expression analysis of terpenoids and pyrethrin biosynthetic pathway of Chrysanthemum cinerariaefolium under abiotic elicitation
[J].
Specific regulation of pyrethrin biosynthesis in Chrysanthemum cinerariaefolium by a blend of volatiles emitted from artificially damaged conspecific plants
[J].
Draft genome of Tanacetum cinerariifolium, the natural source of mosquito coil
[J].
Preparative supercritical fluid extraction of pyrethrin I and II from pyrethrum flower
[J].
Separation and quantitative analysis of natural pyrethrins by high-performance liquid chromatography
[J].
Supercritical fluid extraction and fractionation of natural matter
[J].
Extraction and determination of pyrethrins from pyrethrum cultivated in Iran
[J].
Comparative extraction and enrichment techniques for pyrethrins from flowers of Chrysanthemum cinerariaefolium
[J].
Pyrethrin exraction from pyrethrum flowers using carbon dioxide
[J].
Ultrasound-assisted extraction of pyrethrins from pyrethrum flowers
[J].
Supercritical methodologies applied to the production of biopesticides: a review
[J].
A large-scale application of tissue culture: the mass propagation of pyrethrum clones in ecuador
[J].
Constituents of Chrysanthemum cinerariaefolium in leaves, regenerated plantlets and callus
[J].
The production of pyrethrins by plant cell and tissue cultures of Chrysanthemum cinerariaefolium and tagetes species
[J].
In vitro manipulations for value addition in potent herbal insecticidal activities of Chrysanthemum cinerariaefolium
[M]//KUMAR N.
Trends in chrysanthemic acid chemistry: a survey of recent pyrethrum syntheses
[J].
Nuclear magnetic resonance spectra of the natural pyrethrins and related compounds
[J].
Algae as potential feedstock for the production of biofuels and value-added products: opportunities and challenges
[J].
Complete biosynthesis of opioids in yeast
[J].
梭菌正丁醇代谢工程研究进展
[J].
Recent advances in metabolic engineering of clostridia for n-butanol production
[J].
Production of the antimalarial drug precursor artemisinic acid in engineered yeast
[J].
Synthetic biology tools to engineer microbial communities for biotechnology
[J].
Development of “purple endosperm rice” by engineering anthocyanin biosynthesis in the endosperm with a high-efficiency transgene stacking system
[J].
Versatility in acyltransferase activity completes chicoric acid biosynthesis in purple coneflower
[J].