The increasing emission of CO2 has resulted in severe climate problems, prompting global actions to reduce CO2 emission or fix the atmospheric CO2. In 2020, China set targets for carbon peaking and carbon neutrality, making it an urgent need to develop carbon-fixation technologies. Attributed to the rapid emergence of synthetic biology in recent years, CO2 biotransformation through biochemical reactions catalyzed by enzymes and microbes has achieved a series of significant progress, in the design and engineering of enzymes, metabolic pathway, as well as the construction of in vitro/vivo systems. Many products, such as fuels, amino acids, starch, single-cell proteins, bio-based plastics, and other biocommodities, have been synthesized. Consequently, CO2 is considered as the resource for third-generation biomanufacturing. The crucial step in CO2 biotransformation is the activation of CO2 molecules through the introduction of external energy. Compared to light, heat, and chemical energy, electrical energy is favored due to cost effectiveness, miniaturized apparatus, and convenience, attracting significant attention from both academia and industry. Electrical energy can be utilized in two ways for CO2 biotransformation. In one way, CO2 is electro-activated directly and biotransformed. In the other way, electrical energy facilitates the production of C1 intermediate such as formate, carbinol, CO, and the C1 intermediates are then transformed by coupled microorganisms or enzymes, or the production of reducing forces such as NADH and H2, which participate essentially in CO2 biotransformation. This review comprehensively introduces research advancements in both approaches, analyzes potential carbon-fixation mechanisms, and discusses the advantages and disadvantages of different methods. Furthermore, the review proposes potential synthetic biology strategies to address efficiency concerns in CO2 biotransformation, such as mining highly active carbon-fixing enzyme, enzyme engineering to improve the electron transfer efficiency between the enzyme and the electrode, metabolic engineering to enrich products of carbon-fixing microorganisms and improve the carbon-fixing efficiency, aiming to enable practical applications and the achievement of carbon neutrality goals.
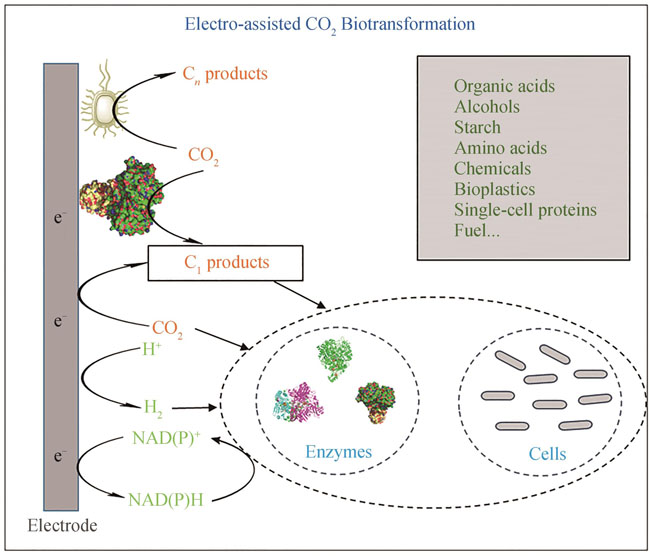