The goals of this article are in memory of Professor Daniel I.C. Wang's contribution to biorefining, the interaction and motivation between Professor Wang and me in this area, and I present opinions on addressing several key challenges of the next-generation biorefinery. Prof. Wang was the primary driver of innovation in both education and multidisciplinary research initiatives that have defined modern Biochemical Engineering, a frontier of chemical engineering. To meet great needs, he adjusted his scientific research directions timely throughout his career from microbial fermentation, single cell protein production, animal cell cultures to biorefineries. The industrial processes of the first-generation biorefinery plants based on food resources (e.g., corn kernel, sucrose, aged grains, used oil) have been very mature, but they potentially have negative impacts on food security and the environment, resulting in their limited development. Due to current low prices of crude oil, the second-generation biorefineries based on non-food lignocellulosic biomass are suffering from relatively high production cost, leading to their economic inviability. New biorefineries, which could be distinctive from the second generation biorefineries that produce liquid biofuels as dominant products, would convert lignocellulosic feedstock (e.g., rice straw, corn stover, energy crops) to multiple products, such as food and feed, healthy sweeteners, and other biocommodities (e.g., biofuels and biomaterials). New biorefineries could be implemented with newly-developed biochemical engineering tools, such as consolidated bioprocessing, novel enzymes, metabolic engineering, in vitro synthetic biology (i.e., multiple-enzyme molecular machines that assemble a number of natural enzymes, novel-function or engineered enzymes, coenzymes, and/or biomimetic coenzymes to form artificial pathways to implement novel biomanufacturing capacity beyond the limits of microbial fermentation and cell cultures). They could be industrial scalability, economic viability, and environmental sustainability. Also, agricultural revolution would take place by the cultivation of perennial plants in marginal lands to replace annual crops, wherein perennial plant communities have higher biomass yield per hectare with less resource management, store more carbon, maintain better water quality, utilize fertilizers more efficiently, tolerate extreme weather, and resist pests. The combination of the cultivation of nonfood perennial (energy) crops and next-generation biorefineries could address major social and economic needs of China, such as food supply, energy security, general health, and environmental conservation. Also, new biorefineries and new agricultural revolution could address challenge of our time to meet increasing need in the energy-food-water nexus without compromising the benefit of next generations.
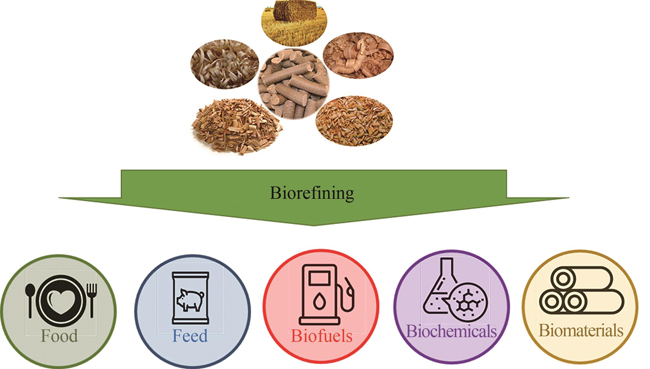