Bone defects caused by trauma, tumors or congenital diseases seriously affect the physical and mental health of human beings. Autologous bone and allogeneic bone transplantation are the gold standard in clinical treatment, but they have certain limitations due to their limited sources, risks of immune response and infection. In recent years, with the improvement of medical technology and cognitive level, the treatment concept of bone tissue defect has gradually changed from tissue transplantation to tissue regeneration mode. Three-dimensional (3D) porous scaffolds play a key role in bone tissue engineering research, serving as biological carriers for seed cells to survive before forming tissues, providing a space for tissue regeneration. The ideal bone tissue scaffold should have good biocompatibility, a porous structure which is conducive to the growth and differentiation of bone cells, suitable mechanical properties, and matching degradation properties. Successful 3D scaffold material design requires understanding the composition and structure of natural bone tissue, selecting appropriate biomaterials, and controllably constructing 3D porous structures at multi-scales through certain fabrication techniques. Natural bone tissue is a composite material with a hierarchical porous structure, which is composed of dense cortical bone in the outer layer and porous cancellous bone in the inner layer. From the perspective of bionics, the regulation of porous scaffolds at multiple scales is an important link in mimicking the hierarchical structure of bone tissue. Silk fibroin (SF), as a natural protein material with good biocompatibility and biodegradability, excellent mechanical properties and easy processing, has become an excellent candidate for the construction of 3D porous scaffolds. This paper summarizes and discusses the research progress of the construction of 3D porous scaffolds based on SF biomaterials. First of all, the characteristics of the multi-layered porous structure of natural bone tissue are summarized; secondly, the composition and structural characteristics of SF materials, as well as their excellent biocompatibility, mechanical properties and biodegradability are described; subsequently, the typical fabrication techniques of SF-based 3D porous scaffolds for bone tissue repair are introduced, including freeze-drying, particle leaching, 3D bioprinting, and composite manufacturing technology, and their ability to control the porous structure of 3D scaffolds, the effects of porous structure on cell growth behavior and bone tissue regeneration are also discussed; finally, the design and fabrication challenges and development prospects of SF-constructed bone tissue repair scaffolds are prosposed, emphasizing that synthetic biology could provide a powerful tool for solving the problems existing in the application of SF-based porous scaffolds in the field of bone tissue engineering.
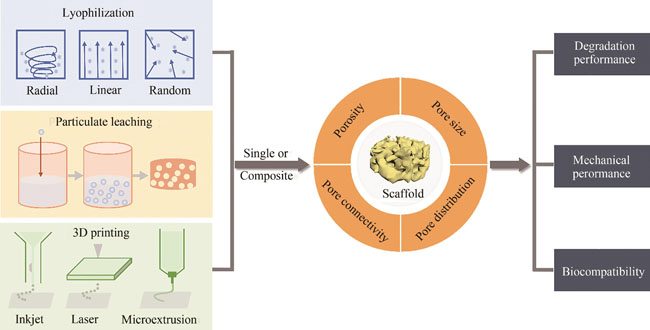