The brain, as the epicenter of human intelligence, sensation, and motor coordination, represents the pinnacle of biological complexity. Despite its critical role, the availability of live human brain tissue for research is fraught with challenges, impeding advancements in our understanding of the nervous system. Brain organoids are sophisticated three-dimensional cultures derived from human pluripotent stem cells that emulate the diverse cellular composition, structural intricacies, and functional attributes of the human brain. These organoids eclipse traditional two-dimensional cultures and animal models in mirroring the brain’s spatial organization and cellular interplay, bolstered by a genetic congruence with their human counterparts. This congruence renders them particularly adept at modeling neuropsychiatric conditions and pioneering cell-based therapeutic interventions. Regenerative medicine, a confluence of engineering and biological sciences, endeavors to restore tissues and organs compromised by aging, disease, or trauma. However, the field grapples with limitations stemming from the scarcity of samples and ethical quandaries. Brain organoid technology emerges as a formidable asset in this domain, offering expansive potential and profound implications for scientific inquiry. Recent strides have seen the successful assembly of organoid models representing various brain regions through the application of tissue engineering and directed differentiation. These models hold promise for simulating neuropathological states and facilitating tissue repair. This article meticulously surveys the cutting-edge methodologies for constructing organoids specific to brain regions such as the cerebral cortex, hippocampus, striatum, midbrain, thalamus, hypothalamus, cerebellum, and retina. It delineates the principal applications of brain organoids in regenerative medicine, encompassing injury simulation, exploration of inter-regional and multi-lineage cellular dynamics, drug efficacy and toxicity assessments, and the potential for organoid transplantation. Furthermore, the review addresses the prevailing obstacles in the application of brain organoids, notably their pronounced variability, absence of vascularization, and developmental immaturity. In essence, this review seeks to illuminate the organoid generation techniques tailored to discrete brain territories and their significance in regenerative medicine’s landscape. By probing into research poised to surmount the limitations of current models, it aspires to broaden the horizons for brain organoids in both foundational research and clinical applications.
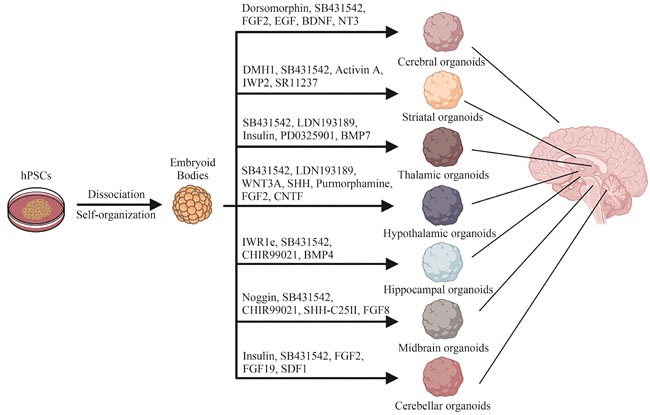